What is the effect of stratospheric aerosol geoengineering on global precipitation? If we were to inject sulphate aerosol into the stratosphere it would reflect some sunlight and cool the Earth, but the atmosphere’s CO2 levels would remain high. This is important, because CO2 actually has an effect on precipitation even when it doesn’t affect surface temperature. In a recent paper with a summer student, I’ve shown the aerosols can contribute a similar effect.
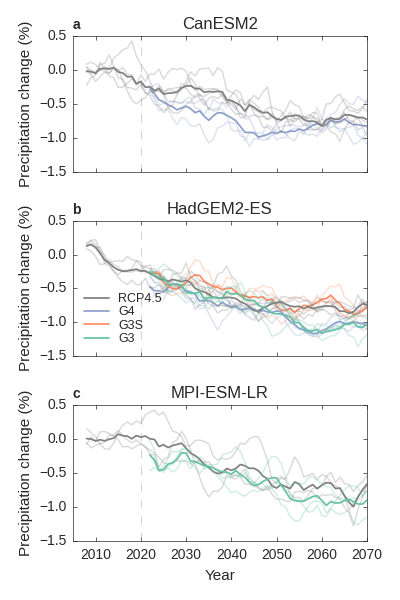
Three climate models (CanESM2, HadGEM2-ES, MPI-ESM-LR) did simulations of the future with and without geoengineering. The simulations with stratospheric aerosols (G3 and G4) show greater temperature-independent precipitation reductions than the simulations without them (RCP4.5 and G3S).
Precipitation as energy flow
Precipitation transfers energy from the Earth’s surface to its atmosphere. It takes energy to evaporate water from the surface. Just as evaporation of sweat from your skin cools you off by taking up heat from your skin, evaporation from the Earth’s surface cools it through energy transfer. Precipitation occurs when this water condenses out in the atmosphere. Condensation releases the heat energy stored when the water evaporated, warming the atmosphere. Globally, precipitation transfers about 78 Watts per square metre of energy from the surface to the atmosphere. Multiplying that by global surface area that’s a total energy transfer of about 40 petajoules (that’s 40 with 15 zeros after it) of energy every second! To put that in a bit of context, it’s about 40% of the amount of energy the Sun transfers to the Earth’s surface.
If precipitation changes, that’s the same as saying the atmospheric energy balance changes. If we warm the atmosphere up, it is able to radiate more energy (following the Stefan-Boltzmann law). To balance that, more energy needs to go into the atmosphere. This happens through precipitation changes.
Direct effects of gases on precipitation
Now imagine we change the amount of CO2 in the atmosphere. This decreases the amount of energy the atmosphere emits to space, meaning the atmosphere has more energy coming in than out. To restore balance the atmospheric heating from precipitation goes down. This means that the global precipitation response to global warming from increasing CO2 has two opposing components: a temperature-independent effect of the CO2, which decreases precipitation, and a temperature-dependent effect which arises from the warming the CO2 subsequently causes. In the long run the temperature-dependent effect is larger. Global warming will increase global precipitation – although there could be local increases or decreases.
But what happens if we do geoengineering? Say we get rid of the temperature-dependent part using aerosols to reduce incoming solar radiation. The temperature-independent effect of CO2 remains and global precipitation will go down.
Detecting the effect of stratospheric aerosol
CO2 isn’t the only thing that has a temperature-independent effect. Any substance that modifies the energy balance of the atmosphere has one. In our new study, we ask whether stratospheric sulphate aerosol has a detectable effect on global precipitation. Theoretically it makes sense, but it is difficult to detect because usually there are temperature-dependent effects obscuring it.
We used a common method to remove the temperature-dependent effect. We calculated the precipitation change for a given surface temperature change from a separate simulation, then used this to remove the temperature-dependent effect in climate model simulations of the future. We did this for future scenarios with and without geoengineering.
As expected, we found a temperature-independent influence which reduced precipitation. Importantly, this effect was bigger when geoengineering aerosols were present in the stratosphere. This was detectable in three different climate models. The figure above shows this. The non-geoengineered ‘RCP4.5’ simulation shows a precipitation decline when the temperature effect is removed. This comes mainly from the CO2. The ‘G3’ and ‘G4’ geoengineering simulations (blue and green lines) have an even greater decline. The aerosol is acting to decrease precipitation further.
How does aerosol affect precipitation?
The temperature-independent effect wasn’t present when geoengineering was done by ‘dimming the Sun’. The ‘G3S’ simulation (orange lines in the figure) does this, and it has a similar precipitation change to RCP4.5. So what causes the precipitation reduction when stratospheric aerosols are used? We calculated the effect of the aerosol on the energy budget of the troposphere (where the precipitation occurs). We separated this in two: the aerosol itself, and the stratospheric warming that occurs because of the effect of the aerosol on the stratosphere’s energy budget.
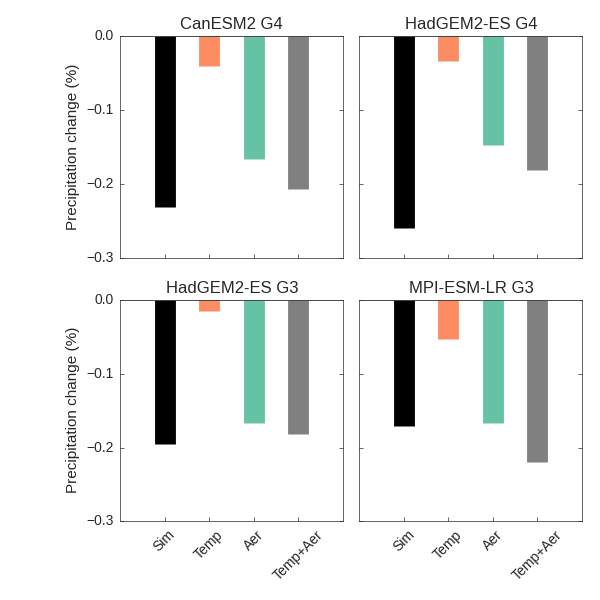
Black bars show the temperature-independent precipitation changes simulated by the models. Orange bars show our calculation of the effect of the stratospheric warming. Green bars show our calculation of effect of the aerosol itself. Grey bars show our calculation of the total effect, which is very close to the actual simulated result.
We found the main effect was from the aerosol itself. The aerosol’s main effect is to reduce incoming solar radiation and cool the surface. But we showed it also interferes a little with the radiation escaping to space, and this alters the energy balance of the troposphere. The precipitation has to respond to these energy balance changes.
This effect is not huge. We had to use many model simulations of the 21st Century to detect it above the ‘noise’ of internal variability. In the real world we only have one ‘simulation’, so this implies the temperature-independent effect of stratospheric aerosol on precipitation would not be detectable in real-world moderate geoengineering scenario. This also means climate model simulations not including the effects of the aerosol could capture much of the effects of geoengineering on the global hydrological cycle.
This effect could be more important under certain circumstances. If geoengineering was more extreme, with more aerosol injected for longer, precipitation would decrease more. But, based on these results, the main effect of geoengineering on precipitation is that the temperature-dependent changes are minimised. This means the temperature-independent effect of increasing CO2 concentrations is unmasked, reducing precipitation.
Take a look at the paper for more details – it’s open access!
Ferraro, A. J., & Griffiths, H. G. (2016). Quantifying the temperature-independent effect of stratospheric aerosol geoengineering on global-mean precipitation in a multi- model ensemble. Environmental Research Letters, 11, 034012. doi:10.1088/1748-9326/11/3/034012.
On a personal note, this paper is significant because it is the culmination of the first research project I truly led. Of course I managed my own research as a PhD student and post-doc, but my supervisors secured the funding. They also acted as collaborators. Here I came up with the idea, applied for funding, supervised Hannah (the excellent student who did much of the analysis) and wrote up the results. It’s a milestone on the way to becoming an independent scientific researcher. For this reason this work will always be special to me. Thanks also to Hannah for being such a good student!